
Quantum Computing
For my English class, I wrote a literature review about the future of quantum computing. It was enthralling to delve into concepts such as superposition, quantum entanglement, and decoherence. For my research, I had the opportunity to contact Dr. Terry Rudolph, who wrote Q is for Quantum, a book that I found integral to my understanding of quantum mechanics. I also communicated with Dr. Scott Aaronson, Director of the Quantum Information Center at the University of Texas, and interviewed one of his students. I learned about their fascinating research to unlock the tremendous promises of quantum computers and had a glimpse into the journey of an aspiring academic researcher.
You can read my review below.
Last Summer, I attended a Summer class at UMass Amherst where I learned how to code in Python exploring concepts used by physicists such as fractals. Then a couple weeks later, I was introduced with real Quantum Computing taught by Qubit by Qubit, coding on the cloud Google Quantum computer!
What is Quantum Computing and Will it Impact our Society in the Future?
By Elois Chadel - December 2023
Abstract
Since the early 1900s, quantum mechanics, the study of microscopic particles and their physical characteristics, has led to major technological advances that have profoundly impacted our life. The most anticipated technological revolution directly resulting from quantum mechanics is the development of quantum computers. These are expected to overshadow classical computers and enable major discoveries in a wide range of fields to significantly impact our society. The aim of this study is to explore the concepts at the basis of quantum physics and evaluate the current state of development of quantum computing. The review of publications, videos and interviews of experts has highlighted the numerous strengths and limitations of this technology. The field of quantum mechanics remains young with entirely new concepts emerging over the past century. While the field evolves at a fast pace, many theoretical and practical aspects remain unknown and scientists need to tackle major limitations before quantum computing becomes practical. This study has uncovered highly divergent opinions about the potential of quantum computers raising the question as to whether exaggerated expectations and unjustified hope were associated with this technology. Implication for future studies includes initiatives to raise public awareness and have an open debate about the risks and prospects of quantum computing, as well as efforts to promote the formation of young physicists who will continue transforming this field.
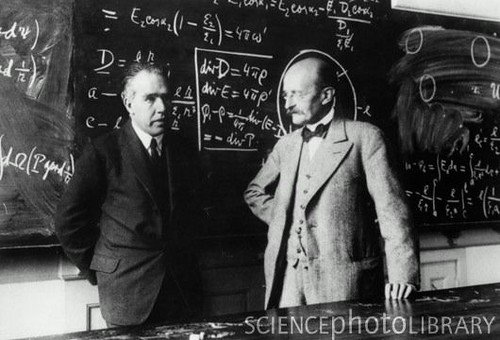
Neils Bohr and Max Planck
Introduction
Quantum computing has been glorified by the media as the next revolutionary level of information technology that will transform the future of scientific research. Ever since Max Planck and Neils Bohr postulated the bases of quantum theory, generations of physicists have leveraged the unusual properties of matter to develop new technologies.
They discovered that matter can have both particle-like and wave-like properties and defined quantum as a discrete quantity (or bundle) of matter. Quantum computing has been glorified by the media as the next revolutionary level of information technology that will transform the future of scientific research. Ever since Max Planck and Neils Bohr postulated the bases of quantum theory, generations of physicists have leveraged the unusual properties of matter to develop new technologies. They discovered that matter can have both particle-like and wave-like properties and defined quantum as a discrete quantity (or bundle) of matter. With quantum theory new mind-blowing concepts emerged such as “superposition”, where different versions of the same particle can exist in different places and states simultaneously, and “entanglement” where two or more particles are intertwined. Quantum physics introduced probability as a fundamental parameter breaking the laws of classical physics. In the past few decades, quantum mechanics has become a vital part of our society. Among the most promising technological advances arising from quantum mechanics is the development of quantum computers. Quantum computers are a variation of information technology that use quantum mechanics to function. Their potential to completely outshadow, and ultimately replace, their predecessors, classical computers, is immense. Indeed, quantum computers are expected to outperform classical computers in speed to an extent that is difficult to comprehend. When available, quantum computers may have numerous applications and there is tremendous hope that they will foster breakthroughs in various areas such as biology, medicine and finance.
However, the frenzied excitement surrounding quantum computers, resulting in massive investments worldwide, has led to much controversy. Indeed, the field is still at its infancy and major limitations need to be overcome for quantum computers to become a viable technology. Some scientists warn about the dangers of making promises that may never be achieved. The purpose of this review is to outline the major concepts of quantum mechanics and to determine both the limitations and the potential of quantum computers in profoundly impacting our society. The methods used for literature research and communication with experts will be presented followed by three parts: 1) an historical view of the major concepts underlying quantum mechanics, 2) a definition of quantum computing and the current state of its development, and 3) a discussion of the controversy that emerged about the future of quantum computing stemming from a limitless potential hindered by still unresolved limitations.

What is Quantum Computing and Will it Impact our Society in the Future?
Quantum Mechanics
Numerous physicists contributed to the birth of quantum theory such as Plank, Bohr, Einstein, Schrodinger, and more (Carroll 2020). Quantum theory tries to explain phenomena happening beyond the macroscopic world and what truly constitutes matter (Norton 2001). In the nineteenth century physicists thought of matter as being either particle-like (such as electrons, protons and neutrons) or wave-like (such as light) (Carroll 2020). However, quantum theory would shatter this neat division of matter by showing that both ordinary matter and radiative matter have both properties, wave and particle. It all started in 1900, when Max Plank in Berlin studied black body radiation (Norton 2001). Through this experiment he created the Planck's constant, a fundamental value in quantum mechanics which is as important in quantum theory as the speed of light is in the theory of relativity established by Einstein in 1905 (Norton 2001). The same year Einstein also proposed that light is made of bundles of energy called “quanta of energy” (Einstein 1905). They were later called “photons” and their recognition was so revolutionary that Einstein won the Nobel prize in 1921 for this work. In 1909, Einstein also furthered Planks research in radiation. He showed that certain phenomena in heat radiation could only be explained by using both waves and particles. This theory called “wave-particle duality” was a crucial step in the field of quantum mechanics (Norton 2001). Around the same time, one of the fundamental theories of particles – the Rutherford Atom – was being put into question. Indeed, Neils Bohr proved that if Rutherford's model was correct then the electrons of an atom would collapse into the nucleus, theoretically ending its existence in a split moment. In 1913, Bohr’s theory posited that there are stable orbits circling the nucleus that allow electrons to orbit without losing energy (Bohr, 1913). Electrons can jump up and down between these allowed orbits by using energy of the light that is transferred to the electron. It even adhered to Plank’s formula (Carroll, 2020; Norton, 2001). Finally, in the middle of the 1920s, Heisenberg, Born, Jordan, de Broglie, and Schroedinger all separately came up with “the new quantum theory”. This theory concluded that matter is made of a combination of both particles and waves, and introduced an element of probability that was unheard of in classical physics (Norton, 2001).
Quantum mechanics theory is often related back to thought experiments: a method that pushes the scientist out of their comfort zone and allows them to confront obstacles that are not easily surpassed. A thought experiment that was essential to explain quantum mechanics was brought to light by Erwin Schrodinger in 1935 in a discussion of an article by Einstein, Rosen and Podolsky (Einstein et al, 1935). Schrodinger’s goal was to describe the probabilistic world of quantum mechanics through the world of classical mechanics that we live in (World of Physics, 2001). The experiment consists of a cat, a box, a radioactive atom, and a vial of hydrocyanic acid connected to a Geiger counter. There is a 50:50 chance that the radioactive atom will decay, trigger the Geiger counter, and break the vial of poison, killing the cat. According to the world of classical physics, where everything is decided through cause and effect, the cat is either dead or alive before the box is opened. On the other hand quantum theory states that the cat is neither dead or alive, but a superposition of both states. In the quantum world, all of the possible products exist simultaneously, and only once they are observed will they collapse into a singular outcome (World of Physics, 2001). This mind-blowing principle of quantum mechanics is called superposition, and has been proven through real-life experiments in the late 1900s. In 1996, at the National Institute of Standards and Technology in Boulder, Colorado, physicists experimented on the eigenstates of a beryllium atom (Monroe et al, 1996). They successfully observed two separate versions of the atom in different places and states simultaneously (Monroe et al, 1996).
Notably, quantum theory was not accepted at once. In 1935, Einstein, Rosen, and Podolsky published a joint paper titled “Can Quantum-Mechanical Description of Physical Reality be Considered Complete?” which discussed their skepticism about quantum mechanics being a complete description of reality (Einstein et al, 1935). A fundamental property of quantum mechanics was introduced in this paper: quantum entanglement. Quantum entanglement is a case in which two or more particles are intertwined so that their quantum state has to be described with reference to each other. Position, momentum, spin, and polarization are all interconnected for a pair of entangled particles, no matter the distance (Vergos, 2021). An example of this could be shown through two theoretically entangled pennies. If one of the coins lands heads, then the other–regardless of distance–will land tails (Gerson, 2018). Also, this phenomena seemingly breaks the laws of classical physics. A set limit in the universe is the speed of light because nothing can travel beyond it, including information. At odds with this, entanglement somehow transfers the information that the first penny landed heads so that the other penny will land tails. This shocked every physicist of the early 1900s, especially Einstein, leading him to even call it a “spooky action at a distance” (Einstein et al, 1935). Later on, it was further researched by physicists Drs. Alain Aspect, John F. Clauser, and Anton Zeilinger, who won the 2022 Nobel Prize in physics for their research in quantum entanglement and quantum information (Johnston, 2022). Up until now, quantum entanglement has been at the basis of countless advancements and experiments in science, the most notable being quantum computing (Vergo, 2021).
In the history of physics, there have been many milestones such as the beginning of classical mechanics, the discovery of relativity, and the emergence of quantum mechanics. With new technologies constantly emerging such as lasers, MRI scanners, and GPS, the world is rapidly evolving. Quantum theory has been and will continue to be at the core of modern society.

Quantum Computing
In the article “Quantum Computing: What It Is, Why We Want It, and How We’re Trying to Get It” by Sara Gamble (2019), quantum computing is introduced as a branch of computer science where quantum properties are used to store information inside subatomic particles. Quantum physics and information theory were first introduced by Dr. Richard Feyman in 1982 at the Massachusetts Institute of Technology (MIT) computer science and Artificial Intelligence Laboratory. The initial idea was to create a computer that could describe the interactions of quantum particles on an accurate scale, which no other computer had been able to do before. The topic did not attract that much attention until 1994 when Dr. Peter Shor, a professor of mathematics at MIT, created a groundbreaking quantum algorithm that could factor integers countless times faster than modern computers. If achieved, Quantum computing has the potential to break numerous security systems that are crucial in our current society. For example, most online transactions use RSA cryptography, which rely on the factoring of immense numbers to protect financial deals. Quantum computing could decode encrypted transactions, raising some concerns about its potential applications.
What makes quantum computers stand out are their core differences from their predecessors, classical computers. One of the main differences is the way they store information. Classical computers are composed of bits, sets of 0’s and 1’s that can be turned on or off. The greater number of bits, the greater number of possible combinations. One bit has 2 possible combinations, 8 bits have 256, and 8192 bits (a kilobyte) have 2^8192 possible combinations. Over the past decades the number of bits in our computers have steadily increased, strongly affecting their performance and leading to the omnipresence of computers in our daily life. Quantum computers are made up of quantum bits, or qubits for short. The difference between bits and qubits is that the latter can run a superposition of every possible combination. This property allows qubits to solve problems exponentially faster than bits. For example, 8 qubits would be able to run 256 combinations simultaneously. Once measured, they all collapse into a single state. The problem with this is that for every superposition to collapse into the desired answer, it would require an immense amount of luck. Physicists change this by using other properties of subatomic particles such as quantum entanglement (Giles, 2021). Quantum entanglement is the property in which 2 or more particles, or qubits in this case, are in a state in which they cannot be described alone without referencing the others (Gamble, 2019). By entangling qubits together, it is possible to leverage the probability of a specific answer being measured. Algorithms such as Schor’s algorithm and Grover’s algorithm use both core quantum properties such as superposition and entanglement to control qubits in a way that solves the given problem (Gamble, 2019).
Quantum computers aren’t perfect, and are set back by the unpredictability of quantum mechanics. William Kretschmer–a PhD candidate in Computer Science at the University of Texas Austin advised by famous quantum physicist Dr. Scott Aaronson–is currently researching some of the limitations of quantum algorithms, and although he specializes in the theoretical aspects of quantum computing he believes that the technological side is where most of the limits in the field originate from. There is a very fine line between the microscopic and macroscopic worlds, with quantum properties only applying in the former. The rules of quantum mechanics no longer apply when the system becomes too big. Quantum computational systems are defined in the microscopic realm and sometimes the properties of superposition and entanglement get broken when stepping into the macro realm. In addition, quantum mechanical properties are very fragile and can often shatter due to interference from the outside world (Giles, 2021). This notion raises the importance of working in a completely closed system. Any sort of interference such as vibration or a change in temperature, could affect superposition and entanglement of a qubit, changing the expected answer. This phenomenon is called decoherence, and although engineers spend a lot of time researching how to isolate qubits as efficiently as possible, it is still a massive problem limiting the democratization of quantum computers (Giles, 2021). To alleviate this issue of decoherence, scientists keep quantum computers in refrigerators at temperatures as close as physically possible to absolute zero. The computers themselves are made of superconductors, which at extremely low temperatures have almost no resistance at all to currents. This allows information to travel at unrestrained speeds nearing the speed of light. Other than increasing the efficiency of the computers, bringing the computers to that temperature limits the amount of interference that can affect them. Lower temperatures lessen vibrations, such as sound and heat waves, which become almost nonexistent at temperatures close to absolute zero (Giles, 2021).
The article “How to Fix Quantum Computing Bugs” by Zaira Nazario (2022) addresses another issue faced by quantum computing. Nazario says that quantum computing’s biggest obstacle is quantum computational error. It is inevitable for information technology to have errors, and the only way to ameliorate them is through error correction. The idea of error correction was first introduced by Dr. Peter Shor and Dr. Andrew Steane in 1995 to make quantum computers more reliable. Every qubit has a possibility of giving the wrong result, but Shor and Steane resolved this problem by using separate qubits just to verify the answers of each central qubit. To do this, they entangled several qubits to a central qubit so that any extraneous answers could be eliminated from the equation. The more error correcting qubits attached to each qubit the less possible errors there will be, because of the increased number of variables in the equation. The issue is that to make a single error-less qubit, or a logical qubit, you would need thousands of error correcting qubits entangled to it. A successful quantum computer would have to be able to solve any problem with a negligible error rate which has not yet been achieved successfully (Nazario, 2022).
In summary, quantum information theory was pioneered by Richard Feyman in 1982 with the hope of creating a new superior branch of information technology. Quantum computers are the combination of quantum mechanics and information theory. They store information using qubits rather than bits with a tremendous potential to solve problems much faster. However, quantum computers are still limited by technological hurdles. Quantum mechanical properties are extremely sensitive to interference from the outside world rendering current quantum computers highly impracticable. They are also prone to numerous errors that are not yet controlled by the current methods for error correction. While highly promising, quantum computing remains at its infancy.
What is the Future of Quantum Computing?
Ever since quantum computers have been introduced to the world as having almost limitless potential to advance scientific discoveries, the hype has been massive. Assumptions that a successful quantum computer would cure cancer, get us to Mars, and other similar outlandish theories were spread throughout the internet. Although this did allow the field to gain a lot of funding, it gave the public an unrealistic projection of what it would accomplish. Several scientists including Dr. Scott Aaronson at the University of Texas Austin are concerned that researchers may be making promises they can’t keep (Horgan, 2021). In 2012, Dr. John Preskill, a theoretical physicist working at the California Institute of Technology, coined the term “quantum supremacy” (Horgan, 2021). Some people think that quantum supremacy is the point where quantum computers can do problems that are impossible for classical computers. In reality, the threshold of quantum supremacy is reached when a quantum computer solves a problem that a classical computer cannot solve in a practical amount of time. The reason quantum computers were created was to solve problems faster than their predecessors so supremacy is an imperative turning point. Once quantum supremacy is achieved, paths will open for quantum computing everywhere, and the investments, as big as they already are, will skyrocket (Harnett, 2021).
A fierce race for building a quantum computer has started involving some of the largest companies such as Google and IBM, as well as new startup companies (Harnett, 2020). In 2020 Google built a quantum computer with 53 qubits and claimed that they had achieved quantum supremacy. This computer called the Sycamore quantum computer was able to solve a specific factoring problem in a total of 200 seconds. Although this does not seem impressive alone, according to Google the strongest classical supercomputer (the IBM Summit Machine) would take 10,000 years to complete the exact same question. IBM disproved this a few days later, showing that the IBM Summit Machine could solve the problem in 2 and a half days. Nevertheless, the performance of the Sycamore quantum computer represents a milestone in achieving quantum supremacy since 2 and a half days necessary for the classical computer is not a practical amount of time. With only 53 qubits, a quantum computer finished a problem over 1000 times faster than the fastest supercomputer in the world. Presently, the quantum computer with the most qubits built into it is the IBM Osprey that has 433 qubits. Smaller companies have also made striking progress including PsiQuantum co-founded in 2016 by Dr. Terry Rudolph. He is convinced that PsiQuantum will have built a useful room-sized quantum computer able to solve impactful problems by the middle of the decade (Horgan, 2021).
As new quantum computers are being built, it remains extremely difficult to demonstrate quantum supremacy. There is so far a very limited practical use for these machines and methods to prove that a computer is faster than another are not well established (Horgan, 2021). Quantum computers may prove to be superior to classical computers only in specialized problems. A certainty is that scientists are “not envisioning quantum computers completely replacing classical computers anytime soon” (Gershon, 2018).
Despite the numerous obstacles, excitement to push forward the development of quantum computing is fueled by the tremendous promise of the technology. It is predicted that quantum computing will allow us to solve problems that are inaccessible so far (Harnett, 2021). There are high expectations about the impact quantum computing may have in influencing numerous fields from health care to climate change by simulating complex phenomena (Horgan, 2021). It is hoped that quantum computers will be powerful tools to simulate quantum systems such as the chemical bonding of complex molecules. Improving our understanding of chemical properties may have a major impact for drug development. Quantum computing is also predicted to transform financial modeling with consequences for the world economy. Another application of quantum computing would be creating more advanced cryptography to enhance security in both military and civil applications (Gamble 2019). A successful quantum computer would be able to create encryptions that are near impossible for a classical computer to break. Alternatively, they would also be able to easily break any encryptions made by classical computers.
Many countries around the world have been attracted by the promise of a revolutionary technology that could replace classical computing in a few decades. Many have tried to get a head start in the field by investing large amounts of money into the research and development of quantum computers. For example, the European Union invested 1 billion dollars into funding research around Europe, and China invested 10 billion dollars in a research facility dedicated to the advancement of quantum information sciences. Quantum computing is not lacking funds, and is set to advance in the near future (Greenemeier, 2018).
Although many people believe that a successful quantum computer is a few years away, history has shown that groundbreaking discoveries such as the first classical microprocessor take decades to appear. For quantum computing to truly succeed, it might take several dozen years before a sufficiently advanced computer is usable. What is sure though is that it is a revolutionary concept that will take the world by storm once it is created, just as classical computers did in the past.
Discussion
Significance of Findings
Quantum computing has been portrayed by the media as the future of information technology. The excitement behind the field is massive, and expectations have built up to an unrealistic level. Although the future of quantum computing is deemed to be vast, its potential is only theoretical, as its practicality remains limited (Horgan, 2021). Quantum computing’s first and foremost obstacle is decoherence, any sort of interference such as vibration or a change in temperature, which could affect the superposition and entanglement of a qubit, changing the expected answer. Many scientists are afraid that quantum computing would not progress due to this property. Scientists have found a solution to this problem called quantum error correction. However, the proposed solution does not successfully overcome the issue since the amount of qubits necessary to create a perfect error correction system is far beyond what is possible today (Nazario, 2022; Giles, 2021). Despite this barrier, scientists have made enormous strides in the field of quantum computing. In this paper, every study reviewed stated that quantum computing had enormous potential, but when that potential would be tapped into was a controversial topic. Some authors thought a complete quantum computer would be created in a few years, while others said it would take decades. History has shown that seemingly impossible tasks–such as creating the first modern computer or successfully modifying genes–take decades to complete, and quantum computing is no different (Kretchner, 2023). Despite the field being very costly, data shows that cost will not be a limiting factor in the near future since billions of dollars have already been invested in quantum computing worldwide (Greenemeier, 2018).
Limitations of Study
One limitation of this study is the complexity of the material. As quantum mechanics is learned about later on in college, the amount a highschool student can elaborate on such an intricate subject is finite. If an undergraduate or graduate student wrote this paper, the research would have been done completely differently, focusing more on actual scientific studies as opposed to researching the knowledge required to understand the topic. Another limitation would be the number of varying theories in quantum mechanics. Depending on the study the findings can be vastly different, mostly due to the differing technology regarding quantum information. Often no consensus is reached among the physicists and many concepts remain theoreticals. Theories are fiercely debated and opinions diverge on the realistic potential of quantum computing in revolutionizing our world. Also, quantum mechanics has extremely convoluted explanations that are difficult to interpret in a simple way. Conveying the tremendous potential but also limitations of quantum mechanics to people is challenging. Another limitation is that only those who work in the field can have credible, though often differing, opinions creating a large bias where not many people can refute the hype building up. This is not a major problem though, because many independent studies have shown the potential of quantum computing and the hype is fueled by concrete and steady advances of the field.
Implications of Further Research
More studies are needed to determine whether or not a quantum computer is faster than a classical computer for a defined task as our current methods are unable to determine this with certainty. For this reason it is not clear when “quantum supremacy” will be attained and what the exact criteria are to achieve this goal. Future research will be necessary to establish standardized methods accepted by the scientific community for comparing emerging technologies with classical computing technologies. While astronomical funds are invested in developing quantum computing it will be important to demonstrate that this area of investigation is not a dead end and can live up to the tremendous expectations. For this, we need more than the often biased opinions of a few extremely specialized scientists and hopeful investors. It would be worthwhile to train more scientists to enter the field of quantum computing to ensure that breakthroughs are made to tackle the current challenges. From this perspective, initiatives to engage students as early as high school to become familiar with the concepts of quantum mechanics will be crucial in fostering the next generation of quantum physicists.
Conclusion
In conclusion, quantum computing could change how science is approached as a whole, but only if scientists are able to surpass the obstacles set by the laws of quantum physics. In view of the rapid evolution of classical computers from slow, complex, room size machines in the fifties to individual laptops and smartphones that have infiltrated all aspects of our life, it is not surprising that the prospect of an even more powerful technology triggers so much hype. Research shows that it will probably take several decades before a practical quantum computer is created. More data is needed to understand how quantum computers stand against supercomputers as they are both designed for different applications. Unfortunately, even in the field of physics, only few researchers focus on developing quantum computers. Concerted efforts are needed to increase the formation and recruitment of quantum physicists who have the expertise required to advance this promising technology.
Bibliography
Bohr, N. (1913). XXXVII. on the Constitution of atoms and molecules. Taylor & Francis.
Caroll, S. (2020) A brief history of quantum mechanics
Giles, M. (2021). Explainer: What is a quantum computer? MIT Technology Review.
Greenemeier, L. (2018). How close are we-really-to building a quantum computer? Scientific American.
Horgan, J. (Scientific American, 2021). Will quantum computing ever live up to its hype?
Norton, J. (Pitt.edu, 2001). Origins of Quantum Theory.
Nazario, Z. (Scientific American, 2022). How to fix quantum computing bugs.